Single-cell metabolomics analysis can provide a lot of help for cell function analysis. However, subject to test conditions, the results obtained have not been satisfactory. With the development of mass spectrometry and single cell extraction methods, a variety of different extraction methods have emerged. This paper briefly describes the new non-destructive extraction testing technology based on FluidFM extraction technology and MALDITOF.
It is well known that due to the existence of cellular heterogeneity, the actual structure and function of each cell are not completely the same, so the study on the single cell level becomes very meaningful. At present, single-cell sequencing and transcription technology have been developed relatively well, with relatively mature models. However, the study of single-cell metabolomics is still in its infancy because the metabolism of cells is very complicated. This is not only a variety of chemical components in the cell, but also a low concentration, and the label is likely to cause its biological function to be disturbed. Therefore, measurement strategies using highly sensitive mass spectrometry have the potential to solve this problem.
In addition to the detection instrument, the extraction means also plays an important role in the detection process. The main means of current extraction is to digest, suspend, and then lyse the cells to obtain the content. This method requires the cells to be suspended and lysed using an enzyme. This process changes the environment in which cells live and will unavoidably have a devastating effect on intracellular metabolites. In addition, changes in individual cells will inevitably have an unknown effect on surrounding cells. Therefore, it is possible to use in situ extraction to extract content without destroying cell structure and vitality, which will greatly help researchers obtain the most original information. However, until now, there is still no ideal way to extract without destroying the cells. This article describes the use of FluidFM technology for single-cell in vivo extraction and parallel mass spectrometry for the detection of metabolites.
Since CELL has previously reported the use of FluidFM technology for in vivo extraction, the authors have developed a set of analytical methods suitable for MALDI-TOF mass spectrometry by studying the author's work, as shown in Figure 1, and confirmed this method. Has a high sensitivity.
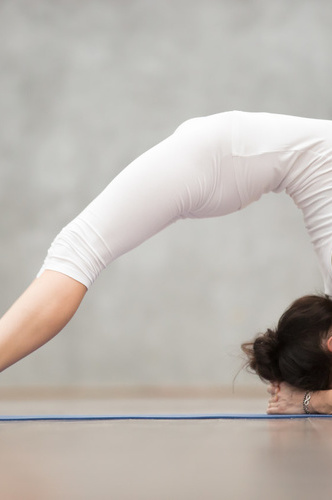
Figure 1. Single cell mass spectrometry analysis using FluidFM and MALDI-TOF. (A) Schematic diagram of single cell extraction; (B) Place the extract in the MAMS well; (C) Spray 9AA matrix to cover the surface; (D) MALDI-TOF mass spectrometry.
The authors successfully extracted 0.8 to 2.7 pL of cytoplasm from pre-used GFP-HeLa cells using a directly purchased 400 nm pore size FluidFM probe, ensuring cell survival on the one hand and sufficient for detection. The cell extract was then placed on a mass spectrometer-specific 95 μm microarrays and immediately blown dry to quench the enzyme activity as shown in Figure 2.
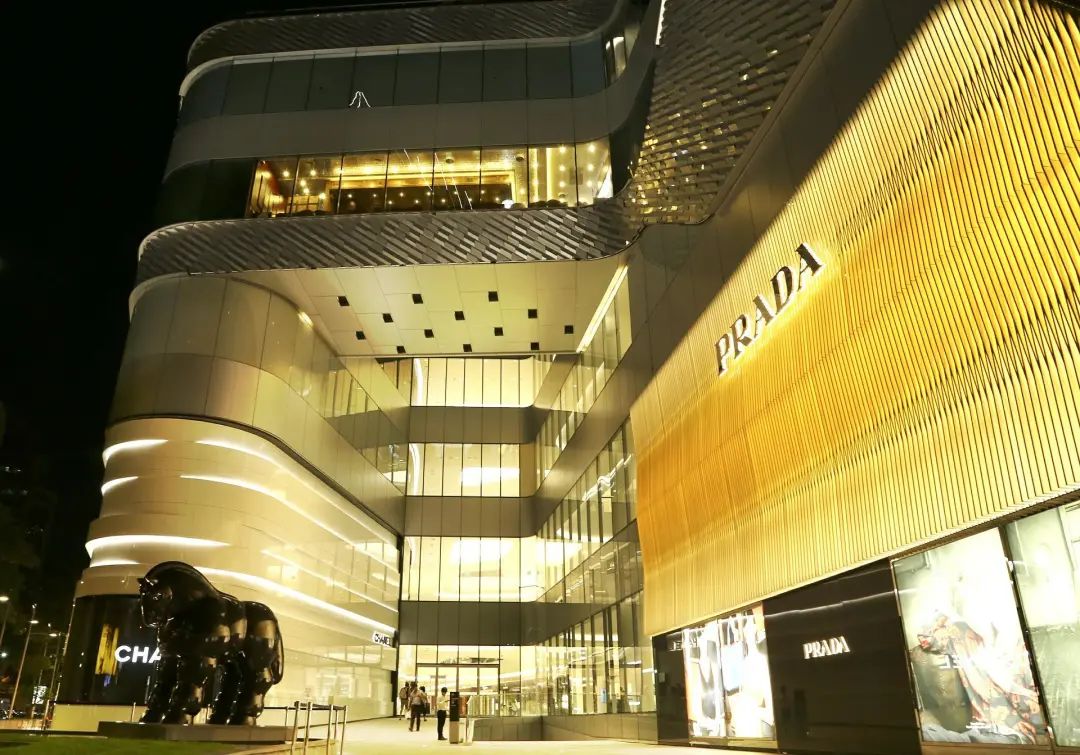
Figure 2. HeLa cell in vivo extraction process. (A) before extraction; (B) extraction; (C) after extraction; (D) cytoplasmic extraction in the probe; (E) movement onto the MAMS well; (F) release of the extract.
9AA was then sprayed onto the microarrays surface and placed in mass spectrometry. The result is shown in Figure 3. They successfully detected various acids and phosphates by this method, including ribonucleotides (cGMP), UDP, ADP, ATP, active sugar (UDP-GlcNAc, UDP-Glc), and amino acids (aspartate). , glutamic acid), glutathione.
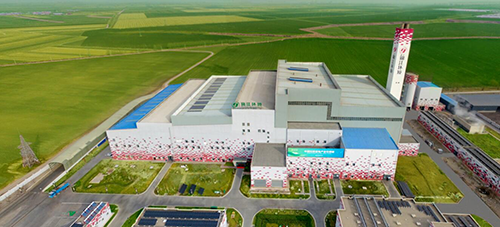
Figure 3. Results of MS analysis of directly extracted HeLa cytoplasmic metabolites.
They then using Glucose C 13 cells were labeled for 48h cells were extracted and detected, as shown in FIG.
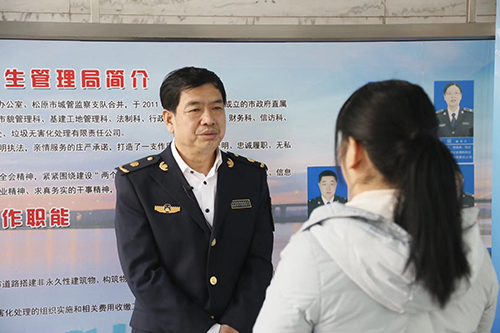
Figure 4. Metabolite results for UC 13 labeled glucose-grown HeLa cells.
In general, the isotope peaks of general compounds in mass spectrometry are often dominated by +1. The main skeleton of U- 13 C has been labeled with C 13 , so the substance with high isotopic accumulation is the main product of metabolism. From the results, the accumulation of isotopes mainly appears on the nucleotides and activated sugars, while glutamic acid and glutathione do not have isotopic accumulation. This indicates that glutamic acid and glutathione are derived from the medium, and glucose synthesizes active sugars and nucleic acids mainly through the sugar Phosphate pathway. As shown in Figure 5.
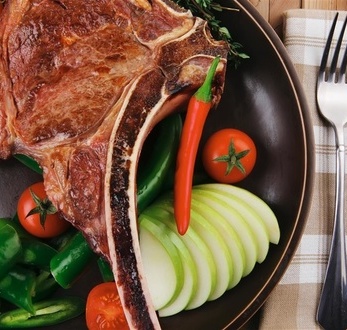
Figure 5. Analysis of U- 13C labeling and natural HeLa metabolites. Arrows indicate peaks of metabolites. * Glutathione peak. ** 13 C UDP GLCNAC [M+13-H]-peak.
to sum up:
In this paper, a new way to directly extract cytoplasm from living cells to study metabolites is realized. In this way, the researcher can study the most primitive metabolic information of the cells without killing the cells and changing the surrounding environment of the cells, and it is possible to continuously monitor the same cells. It is believed that the use of FluidFM technology extraction technology in the near future will help researchers better overcome single cell extraction barriers and allow researchers to obtain more reliable and accurate single cell information.
In this paper, a new way to directly extract cytoplasm from living cells to study metabolites is realized. In this way, the researcher can study the most primitive metabolic information of the cells without killing the cells and changing the surrounding environment of the cells, and it is possible to continuously monitor the same cells. It is believed that the use of FluidFM technology extraction technology in the near future will help researchers better overcome single cell extraction barriers and allow researchers to obtain more reliable and accurate single cell information.
Multifunctional single cell microscopy operating system - Cytosurge FluidFM BOT, Switzerland
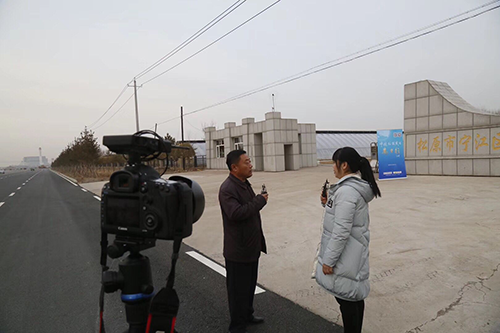
Product introduction:
The versatile single-cell microscopy operating system, FluidFM BOT, is a single-cell operating system that integrates an atomic force system, a microfluidic system, and a cell culture system. The main functions include single cell injection, single cell extraction, and single cell separation.
FluidFM BOT greatly facilitates single-cell level studies, especially for precision medicine, single-cell biology, single-cell mass spectrometry, single-cell gene editing, and drug discovery.
The versatile single-cell microscopy operating system, FluidFM BOT, is a single-cell operating system that integrates an atomic force system, a microfluidic system, and a cell culture system. The main functions include single cell injection, single cell extraction, and single cell separation.
FluidFM BOT greatly facilitates single-cell level studies, especially for precision medicine, single-cell biology, single-cell mass spectrometry, single-cell gene editing, and drug discovery.
Single cell manipulation solution for injection, extraction and sorting
references:
(1) Tang, FC; Lao, KQ; Surani, MA Nat. Methods 2011, 8 (4), S6-S11.
(2) Fessenden, M. Nature 2016, 540 (7631), 153-155.
(3) Zenobi, R. Science 2013, 342 (6163), 1243259.
(4) Pan, ZZ; Raftery, D. Anal. Bioanal. Chem. 2007, 387 (2), 525-527.
(5) Svatos, A. Anal. Chem. 2011, 83 (13), 5037-5044.
(6) Bowen, BP; Northen, TRJ Am. Soc. Mass Spectrom. 2010, 21 (9), 1471-1476.
(7) Heinemann, M.; Zenobi, R. Curr. Opin. Biotechnol. 2011, 22 (1), 26-31.
(8) Amantonico, A.; Oh, JY; Sobek, J.; Heinemann, M.; Zenobi, R. Angew. Chem. Int. Edit. 2008, 47 (29), 5382-5385.
(9) Martano, G.; Delmotte, N.; Kiefer, P.; Christen, P.; Kentner, D.; Bumann, D.; Vorholt, JANat. Protoc. 2015, 10 (1), 1-11.
(10) Tachibana, C. Science 2014, 345, 1519-1521.
(11) Nemes, P.; Knolhoff, AM; Rubakhin, SS; Sweedler, JV Anal. Chem. 2011, 83 (17), 6810-6817.
(12) Nemes, P.; Knolhoff, AM; Rubakhin, SS; Sweedler, JV ACS Chem. Neurosci. 2012, 3 (10), 782-792.
(13) Ong, TH; Kissick, DJ; Jansson, ET; Comi, TJ; Romanova, EV; Rubakhin, SS; Sweedler, JV Anal. Chem. 2015, 87 (14), 7036-7042.
(14) Janson, ET; Comi, TJ; Rubakhin, SS; Sweedler, JV ACS Chem. Biol. 2016, 11 (9), 2588-2595.
(15) Urban, PL; Jefimovs, K.; Amantonico, A.; Fagerer, SR; Schmid, T.; Madler, S.; Puigmarti-Luis, J.; Goedecke, N.; Zenobi, R. Lab Chip 2010 , 10 (23), 3206-3209.
(16) Ibanez, AJ; Fagerer, SR; Schmidt, AM; Urban, PL; Jefimovs, K.; Geiger, P.; Dechant, R.; Heinemann, M.; Zenobi, R. Proc. Natl. Acad. . USA 2013, 110 (22), 8790-8794.
(17) Krismer, J.; Steinhoff, RF; Zenobi, R. Chimia 2016, 70 (4), 236-239.
(18) Masujima, T. Anal. Sci. 2009, 25 (8), 953-960.
(19) Date, S.; Mizuno, H.; Tsuyama, N.; Harada, T.; Masujima, T. Anal. Sci. 2012, 28 (3), 201-203.
(20) Fukano, Y.; Tsuyama, N.; Mizuno, H.; Date, S.; Takano, M.; Masujima, T. Nanomedicine 2012, 7 (9), 1365-1374.
(21) Mizuno, H.; Tsuyama, N.; Date, S.; Harada, T.; Masujima, T. Anal. Sci. 2008, 24 (12), 1525-1527.
(22) Esaki, T.; Masujima, T. Anal. Sci. 2015, 31 (12), 1211-1213.
(23) Gong, XY; Zhao, YY; Cai, SQ; Fu, SJ; Yang, CD; Zhang, SC; Zhang, XR Anal. Chem. 2014, 86 (8), 3809-3816.
(24) Pan, N.; Rao, W.; Kothapalli, NR; Liu, RM; Burgett, AWG; Yang, ZB Anal. Chem. 2014, 86 (19), 9376-9380.
(25) Zhang, LW; Vertes, A. Anal. Chem. 2015, 87 (20), 10397-10405.
(26) Aerts, JT; Louis, KR; Crandall, SR; Govindaiah, G.; Cox, CL; Sweedler, JV Anal. Chem. 2014, 86 (6), 3203-3208.
(27) Guillaume-Gentil, O.; Grindberg, RV; Kooger, R.; Dorwling-Carter, L.; Martinez, V.; Ossola, D.; Pilhofer, M.; Zambelli, T.; Vorholt, JA Cell 2016, 166 (2), 506-516.
(28) Meister, A.; Gabi, M.; Behr, P.; Studer, P.; Voros, J.; Niedermann, P.; Bitterli, J.; Polesel-Maris, J.; Liley, M.; Heinzelmann, H.; Zambelli, T. Nano Lett. 2009, 9 (6), 2501-2507.
(29) Guillaume-Gentil, O.; Potthoff, E.; Ossola, D.; Franz, CM; Zambelli, T.; Vorholt, JA Trends Biotechnol. 2014, 32 (7), 381-388.
(30) Guillaume-Gentil, O.; Potthoff, E.; Ossola, D.; Dorig, P.; Zambelli, T.; Vorholt, JA Small 2013, 9 (11), 1904-1907.
(31) Schmidt, AM; Fagerer, SR; Jefimovs, K.; Buettner, F.; Marro, C.; Siringil, EC; Boehlen, KL; Pabst, M.; Ibanez, AJ Analyst 2014, 139 (22), 5709-5717.
(32) Jeffries, N. Bioinformatics 2005, 21 (14), 3066-3073.
(33) Vermillion-Salsbury, RL; Hercules, DM Rapid Commun. Mass Sp. 2002, 16 (16), 1575-1581.
(34) Kentner, D.; Martano, G.; Callon, M.; Chiquet, P.; Brodmann, M.; Burton, O.; Wahlander, A.; Nanni, P.; Delmotte, N.; Grossmann, J.; Limenitakis, J.; Schlapbach, R.; Kiefer, P.; Vorholt, JA; Hiller, S.; Bumann, D. Proc. Natl. Acad. Sci. USA 2014, 111 (27), 9929-9934 .
(35) Chen, YJ; Huang, XJ; Mahieu, NG; Cho, K.; Schaefer, J.; Patti, GJ Biochemistry 2014, 53 (29), 4755-4757.
(36) Actis, P.; Maalouf, MM; Kim, HJ; Lohith, A.; Vilozny, B.; Seger, RA; Pourmand, N. ACS Nano 2014, 8 (1), 546-553.
(1) Tang, FC; Lao, KQ; Surani, MA Nat. Methods 2011, 8 (4), S6-S11.
(2) Fessenden, M. Nature 2016, 540 (7631), 153-155.
(3) Zenobi, R. Science 2013, 342 (6163), 1243259.
(4) Pan, ZZ; Raftery, D. Anal. Bioanal. Chem. 2007, 387 (2), 525-527.
(5) Svatos, A. Anal. Chem. 2011, 83 (13), 5037-5044.
(6) Bowen, BP; Northen, TRJ Am. Soc. Mass Spectrom. 2010, 21 (9), 1471-1476.
(7) Heinemann, M.; Zenobi, R. Curr. Opin. Biotechnol. 2011, 22 (1), 26-31.
(8) Amantonico, A.; Oh, JY; Sobek, J.; Heinemann, M.; Zenobi, R. Angew. Chem. Int. Edit. 2008, 47 (29), 5382-5385.
(9) Martano, G.; Delmotte, N.; Kiefer, P.; Christen, P.; Kentner, D.; Bumann, D.; Vorholt, JANat. Protoc. 2015, 10 (1), 1-11.
(10) Tachibana, C. Science 2014, 345, 1519-1521.
(11) Nemes, P.; Knolhoff, AM; Rubakhin, SS; Sweedler, JV Anal. Chem. 2011, 83 (17), 6810-6817.
(12) Nemes, P.; Knolhoff, AM; Rubakhin, SS; Sweedler, JV ACS Chem. Neurosci. 2012, 3 (10), 782-792.
(13) Ong, TH; Kissick, DJ; Jansson, ET; Comi, TJ; Romanova, EV; Rubakhin, SS; Sweedler, JV Anal. Chem. 2015, 87 (14), 7036-7042.
(14) Janson, ET; Comi, TJ; Rubakhin, SS; Sweedler, JV ACS Chem. Biol. 2016, 11 (9), 2588-2595.
(15) Urban, PL; Jefimovs, K.; Amantonico, A.; Fagerer, SR; Schmid, T.; Madler, S.; Puigmarti-Luis, J.; Goedecke, N.; Zenobi, R. Lab Chip 2010 , 10 (23), 3206-3209.
(16) Ibanez, AJ; Fagerer, SR; Schmidt, AM; Urban, PL; Jefimovs, K.; Geiger, P.; Dechant, R.; Heinemann, M.; Zenobi, R. Proc. Natl. Acad. . USA 2013, 110 (22), 8790-8794.
(17) Krismer, J.; Steinhoff, RF; Zenobi, R. Chimia 2016, 70 (4), 236-239.
(18) Masujima, T. Anal. Sci. 2009, 25 (8), 953-960.
(19) Date, S.; Mizuno, H.; Tsuyama, N.; Harada, T.; Masujima, T. Anal. Sci. 2012, 28 (3), 201-203.
(20) Fukano, Y.; Tsuyama, N.; Mizuno, H.; Date, S.; Takano, M.; Masujima, T. Nanomedicine 2012, 7 (9), 1365-1374.
(21) Mizuno, H.; Tsuyama, N.; Date, S.; Harada, T.; Masujima, T. Anal. Sci. 2008, 24 (12), 1525-1527.
(22) Esaki, T.; Masujima, T. Anal. Sci. 2015, 31 (12), 1211-1213.
(23) Gong, XY; Zhao, YY; Cai, SQ; Fu, SJ; Yang, CD; Zhang, SC; Zhang, XR Anal. Chem. 2014, 86 (8), 3809-3816.
(24) Pan, N.; Rao, W.; Kothapalli, NR; Liu, RM; Burgett, AWG; Yang, ZB Anal. Chem. 2014, 86 (19), 9376-9380.
(25) Zhang, LW; Vertes, A. Anal. Chem. 2015, 87 (20), 10397-10405.
(26) Aerts, JT; Louis, KR; Crandall, SR; Govindaiah, G.; Cox, CL; Sweedler, JV Anal. Chem. 2014, 86 (6), 3203-3208.
(27) Guillaume-Gentil, O.; Grindberg, RV; Kooger, R.; Dorwling-Carter, L.; Martinez, V.; Ossola, D.; Pilhofer, M.; Zambelli, T.; Vorholt, JA Cell 2016, 166 (2), 506-516.
(28) Meister, A.; Gabi, M.; Behr, P.; Studer, P.; Voros, J.; Niedermann, P.; Bitterli, J.; Polesel-Maris, J.; Liley, M.; Heinzelmann, H.; Zambelli, T. Nano Lett. 2009, 9 (6), 2501-2507.
(29) Guillaume-Gentil, O.; Potthoff, E.; Ossola, D.; Franz, CM; Zambelli, T.; Vorholt, JA Trends Biotechnol. 2014, 32 (7), 381-388.
(30) Guillaume-Gentil, O.; Potthoff, E.; Ossola, D.; Dorig, P.; Zambelli, T.; Vorholt, JA Small 2013, 9 (11), 1904-1907.
(31) Schmidt, AM; Fagerer, SR; Jefimovs, K.; Buettner, F.; Marro, C.; Siringil, EC; Boehlen, KL; Pabst, M.; Ibanez, AJ Analyst 2014, 139 (22), 5709-5717.
(32) Jeffries, N. Bioinformatics 2005, 21 (14), 3066-3073.
(33) Vermillion-Salsbury, RL; Hercules, DM Rapid Commun. Mass Sp. 2002, 16 (16), 1575-1581.
(34) Kentner, D.; Martano, G.; Callon, M.; Chiquet, P.; Brodmann, M.; Burton, O.; Wahlander, A.; Nanni, P.; Delmotte, N.; Grossmann, J.; Limenitakis, J.; Schlapbach, R.; Kiefer, P.; Vorholt, JA; Hiller, S.; Bumann, D. Proc. Natl. Acad. Sci. USA 2014, 111 (27), 9929-9934 .
(35) Chen, YJ; Huang, XJ; Mahieu, NG; Cho, K.; Schaefer, J.; Patti, GJ Biochemistry 2014, 53 (29), 4755-4757.
(36) Actis, P.; Maalouf, MM; Kim, HJ; Lohith, A.; Vilozny, B.; Seger, RA; Pourmand, N. ACS Nano 2014, 8 (1), 546-553.
Propionate Salts
Wuxi Yangshan Biochemical Co.,Ltd. , https://www.yangshanchem.com